Cement is the chief ingredient in cement paste – the binding agent in portland cement concrete (PCC). It is a hydraulic cement that, when combined with water, hardens into a solid mass. Interspersed in an aggregate matrix it forms PCC. As a material, portland cement has been used for well over 175 years and, from an empirical perspective, its behavior is well-understood. Chemically, however, portland cement is a complex substance whose mechanisms and interactions have yet to be fully defined. ASTM C 125 and the Portland Cement Association (PCA) provide the following precise definitions:
- Hydraulic cement: An inorganic material or a mixture of inorganic materials that sets and develops strength by chemical reaction with water by formation of hydrates and is capable of doing so under water.
- Portland cement: A hydraulic cement composed primarily of hydraulic calcium silicates.
Background
Although the use of cements (both hydraulic and non-hydraulic) goes back many thousands of years (to ancient Egyptian times at least), the first occurrence of “portland cement” came about in the 19th century. In 1824, Joseph Aspdin, a Leeds mason took out a patent on a hydraulic cement that he coined “Portland” cement (Mindess and Young, 1981[1]). He named the cement because it produced a concrete that resembled the color of the natural limestone quarried on the Isle of Portland, a peninsula in the English Channel (see Figure 1 and 2). Since then, the name “portland cement” has stuck and is written in all lower case because it is now recognized as a trade name for a type of material and not a specific reference to Portland, England.
Today, portland cement is the most widely used building material in the world with about 1.56 billion tonnes (1.72 billion tons) produced each year. Annual global production of portland cement concrete hovers around 3.8 million cubic meters (5 billion cubic yards) per year (Cement Association of Canada, 2002[2]). In the U.S., rigid pavements are the largest single use of portland cement and portland cement concrete (ACPA, 2002[3]).
Manufacturing
Although there are several variations of commercially manufactured portland cement, they each share many of the same basic raw materials and chemical components. The chief chemical components of portland cement are calcium, silica, alumina and iron. Calcium is derived from limestone, marl or chalk, while silica, alumina and iron come from the sands, clays and iron ore sources. Other raw materials may include shale, shells and industrial byproducts such as mill scale (Ash Grove Cement Company, 2000[4]).
The basic manufacturing process heats these materials in a kiln to about 1400 to 1600°C (2600 – 3000°F) – the temperature range in which the two materials interact chemically to form calcium silicates (Mindess and Young, 1981[1]). This heated substance, called “clinker” is usually in the form of small gray-black pellets about 12.5 mm (0.5 inches) in diameter. Clinker is then cooled and pulverized into a fine powder that almost completely passes through a 0.075 mm (No. 200) sieve and fortified with a small amount of gypsum. The result is portland cement. The Portland Cement Association (PCA) has an excellent interactive illustration of this process on their website.
Chemical Properties
Portland cements can be characterized by their chemical composition although they rarely are for pavement applications. However, it is a portland cement’s chemical properties that determine its physical properties and how it cures. Therefore, a basic understanding of portland cement chemistry can help one understand how and why it behaves as it does. This section briefly describes the basic chemical composition of a typical portland cement and how it hydrates.
Basic Composition
Table 1 and Figure 3 show the main chemical compound constituents of portland cement.
Table 1. Main Constituents in a Typical Portland Cement (Mindess and Young, 1981[1])
Chemical Name | Chemical Formula | Shorthand Notation | Percent by Weight |
---|---|---|---|
Tricalcium Silicate | 3CaO×SiO2 | C3S | 50 |
Dicalcium Silicate | 2CaO×SiO2 | C2S | 25 |
Tricalcium Aluminate | 3CaO×Al2O3 | C3A | 12 |
Tetracalcium Aluminoferrite | 4CaO×Al2O3×Fe2O3 | C4AF | 8 |
Gypsum | CaSO4×H2O | CSH2 | 3.5 |
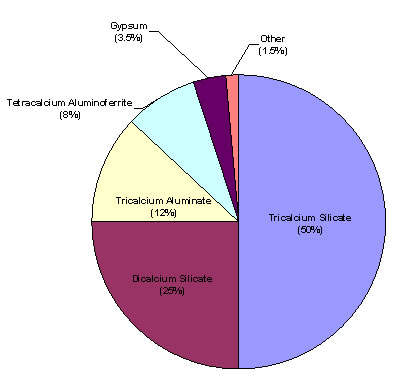
Hydration
When portland cement is mixed with water its chemical compound constituents undergo a series of chemical reactions that cause it to harden (or set). These chemical reactions all involve the addition of water to the basic chemical compounds listed in Table 1. This chemical reaction with water is called “hydration”. Each one of these reactions occurs at a different time and rate. Together, the results of these reactions determine how portland cement hardens and gains strength.
- Tricalcium silicate (C3S). Hydrates and hardens rapidly and is largely responsible for initial set and early strength. Portland cements with higher percentages of C3S will exhibit higher early strength.
- Dicalcium silicate (C2S). Hydrates and hardens slowly and is largely responsible for strength increases beyond one week.
- Tricalcium aluminate (C3A). Hydrates and hardens the quickest. Liberates a large amount of heat almost immediately and contributes somewhat to early strength. Gypsum is added to portland cement to retard C3A hydration. Without gypsum, C3A hydration would cause portland cement to set almost immediately after adding water.
- Tetracalcium aluminoferrite (C4AF). Hydrates rapidly but contributes very little to strength. Its use allows lower kiln temperatures in portland cement manufacturing. Most portland cement color effects are due to C4AF.
Figure 4 shows rates of heat evolution, which give an approximate idea of hydration times and when a typical portland cement initially sets.
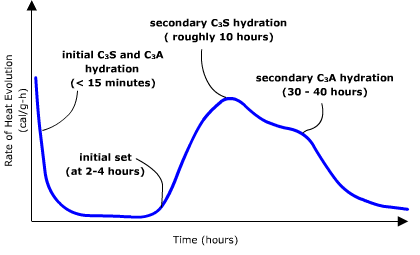
Types of Portland Cement
Knowing the basic characteristics of portland cement’s constituent chemical compounds, it is possible to modify its properties by adjusting the amounts of each compound. In the U.S., AASHTO M 85 and ASTM C 150, Standard Specification for Portland Cement, recognize eight basic types of portland cement concrete (Table 2). There are also many other types of blended and proprietary cements that are not mentioned here.
Table 2. ASTM Types of Portland Cement
Type | Name | Purpose |
---|---|---|
I | Normal | General-purpose cement suitable for most purposes. |
IA | Normal-Air Entraining | An air-entraining modification of Type I. |
II | Moderate Sulfate Resistance | Used as a precaution against moderate sulfate attack. It will usually generate less heat at a slower rate than Type I cement. |
IIA | Moderate Sulfate Resistance-Air Entraining | An air-entraining modification of Type II. |
III | High Early Strength | Used when high early strength is needed. It is has more C3S than Type I cement and has been ground finer to provide a higher surface-to-volume ratio, both of which speed hydration. Strength gain is double that of Type I cement in the first 24 hours. |
IIIA | High Early Strength-Air Entraining | An air-entraining modification of Type III. |
IV | Low Heat of Hydration | Used when hydration heat must be minimized in large volume applications such as gravity dams. Contains about half the C3S and C3A and double the C2S of Type I cement. |
V | High Sulfate Resistance | Used as a precaution against severe sulfate action – principally where soils or groundwaters have a high sulfate content. It gains strength at a slower rate than Type I cement. High sulfate resistance is attributable to low C3A content. |
Physical Properties
Portland cements are commonly characterized by their physical properties for quality control purposes. Their physical properties can be used to classify and compare portland cements. The challenge in physical property characterization is to develop physical tests that can satisfactorily characterize key parameters. This section, taken largely from the PCA (1988[5]), lists the more common U.S. portland cement physical properties that are tested. Specification values, where given, are taken from ASTM C 150, Standard Specification for Portland Cement.
Keep in mind that these properties , in general, apply to “neat” cement pastes – that is, they only include portland cement and water. Neat cement pastes are typically difficult to handle and test and thus they introduce more variability into the results. Cements may also perform differently when used in a “mortar” (cement + water + sand). Over time, mortar tests have been found to provide a better indication of cement quality and thus, tests on neat cement pastes are typically used only for research purposes (Mindess and Young, 1981[1]). However, if the sand is not carefully specified in a mortar test, the results may not be transferable.
- Mindess, S. and Young, J.F. (1981). Concrete. Prentice-Hall, Inc. Englewood Cliffs, NJ.↵
- Cement Association of Canada. (2002). Web site. Accessed at http://www.cement.ca/. Cement Association of Canada. Ottawa, ON. Accessed 15 January 2002.↵
- American Concrete Pavement Association (ACPA). (2002). Web site. Accessed at http://www.pavement.com. Accessed 15 January 2002.↵
- Ash Grove Cement Company. (2000). Web site. Accessed at http://www.ashgrove.com/index.html. Ash Grove Cement Company. Overland Grove, KS. Accessed 15 January 2002.↵
- Portland Cement Association (PCA). (1988). Design and Control of Concrete Mixtures. Portland Cement Association. Skokie, IL.↵