1 Introduction
Growing attention to extreme weather events and the long-term impacts of climate change have begun to focus efforts nationally and internationally on the ability of society and infrastructure to adapt to and recover from future changed conditions and associated disasters. The term resilience has generally come to represent this adaptation/recovery ability. In the last decade, resilience has become a priority consideration in the planning, design, construction, operations, and maintenance of infrastructure.
In the transportation sector, major regional and national organizations have begun in earnest to publish guidance on how scientific climate change predictions can be expected to impact transportation infrastructure and operations. More recently, this guidance has included how resilience can be integrated into infrastructure as a means to address both long-term climate change impacts and short-term extreme events.
Within the pavement industry, momentum is now building to participate (as other industries are) in defining the impacts of climate change and extreme events on pavement structures, assessing identified vulnerabilities, and responding with changes in planning, design, construction, operations, and maintenance. This background document provides an overview of the following:
- Climate change impacts. A general understanding of the scientific consensus on climate change and its impacts is useful in order to understand what may be at risk and what strategies may best address this risk.
- Impacts on transportation systems. There is a subset of climate change impacts of high interest to the transportation sector that can help focus efforts on those that have the highest impact on transportation (including pavements).
- Impacts on pavements. A body of literature is beginning to emerge that describes climate change impacts on pavements and some potential consequences. Currently, work within the community is in the early stages and, as a result, is still exploratory, limited, and local.
- Resilience. The response to climate change impacts is commonly known as resilience. There is a growing body of knowledge that describes systematic approaches to resilience. Such work tends not to address infrastructure as specific as pavements, although it can be used to frame a general pavement resilience approach.
2 Climate Change Impacts
Resilience actions are dependent upon climate change impacts and, specifically, how they might be different than historical climate impacts. This section reviews what those differences are anticipated to be based on national/international summative references.
2.1 Key Summative References
While there are many descriptions of climate change and its impacts, the select summative documents here are a good consensus view of climate change information:
- The Intergovernmental Panel on Climate Change (IPCC) Fifth Assessment Report (AR5) Climate Change 2014 Synthesis Report (IPCC 2014). The IPCC is the U.N. body for assessing the science related to climate change. Starting in 1990, IPCC has produced comprehensive reports every 5 to 7 years and is generally viewed as the global consensus resource on climate change science. The Sixth assessment report synthesis is due out in 2022.
- The U.S. Global Change Research Program’s Fourth National Climate Assessment (USGCRP 2018). The USGCRP is a Federal program codified by Congress in 1990 to coordinate Federal research and investments in understanding the forces shaping the global environment and their impacts on society. Starting in 2000, USGCRP has produced comprehensive U.S. climate assessments every 4 to 9 years and is generally viewed as a national consensus resource on climate change science. The fourth assessment has two main volumes:
- Climate Science Special Report (Volume 1). Reviews the climate science used to predict climate change.
- Impacts, Risks, and Adaptation in the United States (Volume II). Provides climate change details by U.S. region and presents climate change impacts, risks and adaptation issues by national topics (e.g. transportation).
- The National Cooperative Research Program’s NCHRP Report 750: Strategic Issues Facing Transportation, Volume 2: Climate Change, Extreme Weather Events, and the Highway System (Meyer et al. 2014). Slightly more dated than the previous two sources, this report provides an overview of climate change and adaptation for the transportation sector. It contains high-level speculation on pavement impacts, the only reference of these three that does.
2.2 Climate Change Background
Changes in the global climate and the understanding that human activities have been the dominant cause are supported by a preponderance of historical observation and climate modeling both at a national and global scale (IPCC 2014; USGCRP 2018). Current climate models generally predict that the climate will continue to change and do so at an increasing rate over the next century or longer (IPCC 2014; USGCRP 2018). While the magnitude and speed of predicted future climate change is generally dependent upon human activities, even the most optimistic scenarios predict substantial climate change over the next century or longer based on what has already occurred in conjunction with the relatively long life of emitted heat-trapping gases (commonly grouped together as greenhouse gases, or GHG) and slow feedback functions of the atmospheric systems that drive climate change (IPCC 2014). What follows is a selective summary from the USGCRP Fourth National Climate Assessment, Climate Science Special Report (Volume 1 of a 2-volume assessment) of the climate change impacts with the most direct relevance to pavements. Impacts are only included if the USGCRP considered them to be of medium confidence or higher.
Regional impacts. The Fourth National Assessment, Volume II (USGCRP 2018) discuss these impacts in more detail on a region-by-region basis within the U.S. and focuses on 10 defined regions: Northeast, Southeast, U.S. Caribbean, Midwest, Northern Great Plains, Southern Great Plains, Northwest, Southwest, Alaska, and Hawai’i and U.S.-affiliated Pacific Islands.
A note on the uncertainty of climate change. The greatest uncertainty in projecting future climate conditions is the uncertainty in future GHG emissions (USGCRP 2018). To address this uncertainty, the IPCC Fifth Assessment Report (IPCC 2014) does climate modeling over a range of projected future GHG emission scenarios. Each scenario is labeled a representative concentration pathway (RCP) with the suffix reflecting the radiative forcing values, with higher forcing resulting in increased warming, for the year 2100 (see figure 1). Radiative forcing in watts/m2 is not intuitive to most so it may be easier to envision each as a scenario of when GHG emissions peak and then start to decline:
- RCP2.5: Emissions peak around 2010-2020 and then decline thereafter
- RCP4.5: Emissions peak around 2040 and then decline thereafter
- RCP6.0: Emissions peak around 2080 and then decline thereafter
- RCP8.5: Emissions continue to rise throughout the 21st century
Predictions of climate change impacts are dependent upon which RCP is assumed. Some impacts are true for all RCPs (only varying in magnitude: more radiative forcing results in larger impacts) while others are dependent on the RCP assumed such that the prediction may change based on the RCP used. Currently GHG emission trends are consistent with the RCP8.5 scenario.
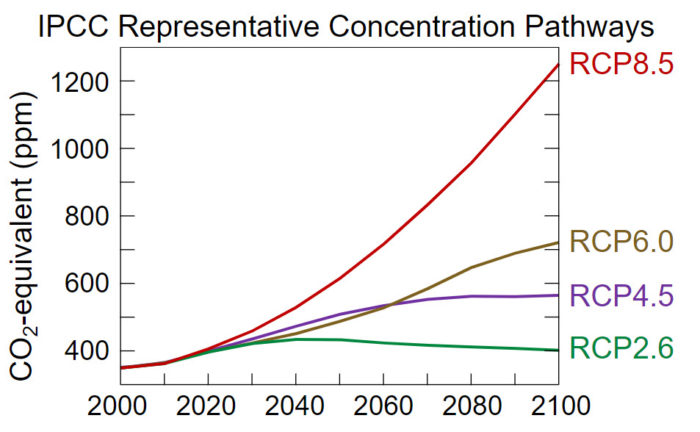
2.3 Temperature
General increase in temperature. Annual average temperature over the contiguous U.S. is projected to rise by about 2.5 °F (1.4 °C) for the period 2021 to 2050 relative to 1976 to 2005 no matter the RCP scenario considered. This is about twice the rate of the previous 50 years. Temperature rises by the late century (2071 to 2100) range from 2.8 to 11.9 °F (1.6 to 6.6 °C) depending upon the RCP scenario (USGCRP 2017). The projected changes in annual temperatures for North America are illustrated in figure 2.
Higher extreme temperatures. Extreme temperatures are predicted to increase even more than average temperatures. Cold waves will become less intense whereas heat waves will become more intense. These changes may also lead to fewer days below freezing, potential changes in the number of freeze-thaw cycles experienced, and reduced frost penetration into soil.
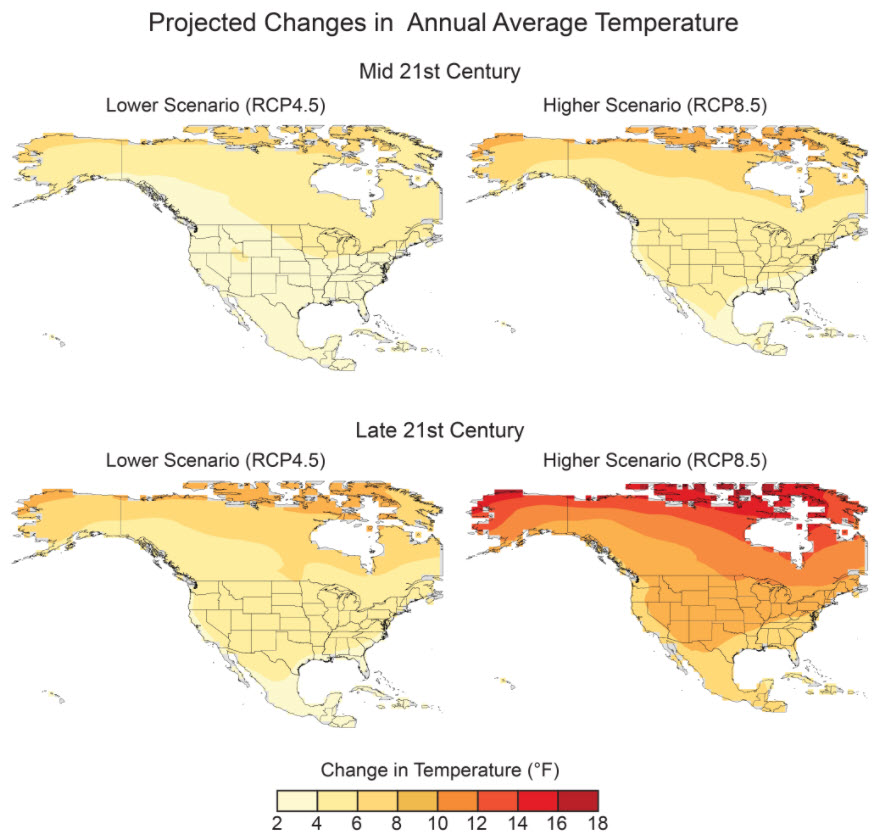
2.4 Precipitation
Changes in annual precipitation will vary across the U.S. Seasonal changes will also occur, with some regions seeing less precipitation (for example, the Pacific Northwest seeing less summer precipitation) while other areas will see more precipitation (for example, the Northeast will see more winter precipitation). Projected changes in seasonal precipitation is illustrated in figure 3.
More frequent and intense heavy precipitation events. Increases are expected to occur for the largest single-day precipitation events. Regional and seasonal differences exist.
Changes in snow patterns. Large declines in snowpack in the west and shifts to more precipitation (less in the form of snow) during the cold season in parts of the central and eastern U.S.
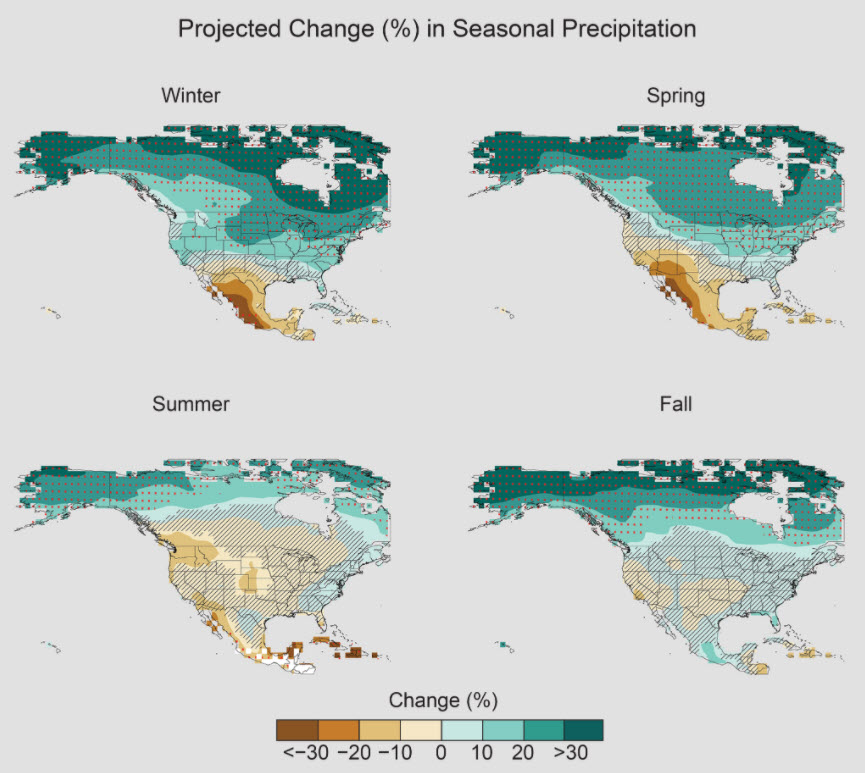
2.5 Droughts, Floods, and Wildfires
Possible increasing chronic, long-duration drought. Under higher RCP scenarios, the potential for an extended drought in some parts of the country is more likely by the end of the century.
Decrease in surface soil moisture. Increased evapotranspiration caused by higher temperatures will reduce soil moisture.
Some classes of flood frequency have changed, but it is difficult to quantify how future flooding may change in response to human-induced climate change. The emergence and timing of any future change in flooding that can be traced to human-induced climate change is unclear.
Increased large forest fire incidences in the western U.S. and Alaska. The annual number of large forest fires have been increasing in number and intensity since 1980. This trend will continue. Figure 4 shows trends in the annual number of large fires in western U.S.
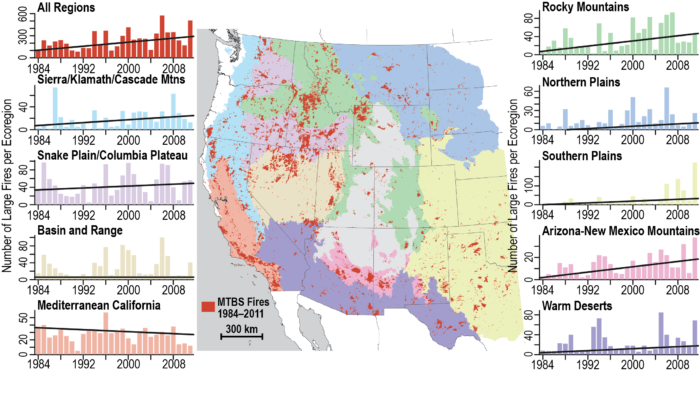
2.6 Extreme Storms
Increase in tropical cyclone intensity and frequency. This is consistent with the observed upward trend in North Atlantic hurricane activity since 1970. Figure 5 shows simulated increases of worldwide tropical storms based on climate change predictions.
Tornado activity has become more variable and confidence in future predictions is low. Models generally predict an increase, but confidence in the details is low.
Projections in winter storm frequency and intensity are varied and prediction confidence is low. Model agreement is poor.
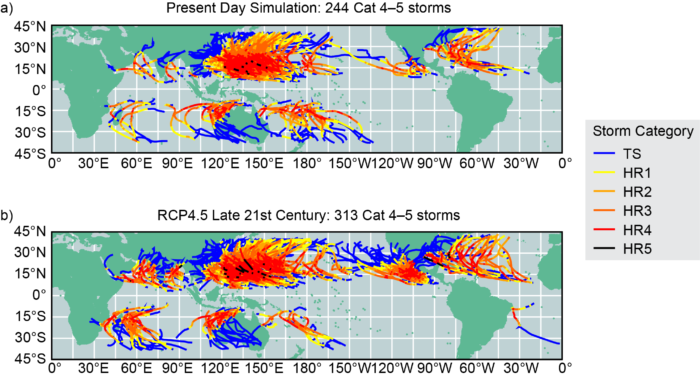
2.7 Arctic Changes
Permafrost in Alaska is thawing and becoming more discontinuous. Air temperatures in Alaska and the Arctic have increased over the last 50 years at a rate of over twice that of the global average.
2.8 Sea Level
Sea-level rise. Global mean sea levels are already rising (7 to 8 inches [178 to 203 mm] since 1900 and about 3 of those inches [76 mm] since 1993) and will continue to do so. Projections on the amount of global mean sea-level rise are in the range of 1.0 to 4.3 ft (0.3 to 1.3 m) by 2100. Rise can vary from location to location based on regional differences in ocean temperatures, salinity, currents, and subsidence or uplift of the coast.
Sea-level rise will increase the depth, frequency, and extent of tidal floods that cause minor impacts. Higher sea levels may result in more flooding issues associated with high tides.
Sea-level rise will increase the frequency and extent of flooding during coastal storms. Higher sea levels may result in more flooding, which may be severe, for a given storm intensity and duration.
3 Impacts on Transportation Systems
Many organizations have begun to plan for climate change, the impacts it may have, and the necessary adaptations needed to minimize or mitigate those impacts. At the highest level, most U.S. government and scientific organizations are in general agreement on the nature of climate change impacts and are beginning to explore how to adapt current practice to account for these impacts. The Fourth National Assessment, Volume II report (USGCRP 2018) describes a wide range of climate change impacts by category and U.S. region. These impacts are many and include, among others, compromised coastal freshwater aquifers, increased electricity consumption, transportation network disruption, declining crop production, increased tree mortality, ecosystem alteration, increased respiratory and cardiovascular disease, and changing land use (USGCRP 2018). For the transportation sector, the report offers the following key messages (USGCRP 2018):
- Climate changes poses a risk to U.S. transportation infrastructure. The impacts from heavy precipitation, flooding, heat, wildfires, sea level rise and storm surge, extreme weather events, Arctic warming, and other climatic conditions are affecting the reliability and capacity of the U.S. transportation system.
- Extreme events can cause strong and varied impacts to urban and rural transportation networks. These impacts can have broad societal and economic consequences and can disproportionately affect vulnerable populations.
- Practitioners are beginning to address climate risks through assessment and adaptive measures. Practitioners are beginning to address climate risks through assessment and adaptive measures. Tools are becoming more widely available that provide guidance and assistance in addressing climate risks but there is much work still to be done to arrive at more robust system-wide approaches.
Within this broad overview some pavement topics are mentioned but only as examples. This lack of detail is common at the national level even among more focused agency-level efforts (e.g. table 1).
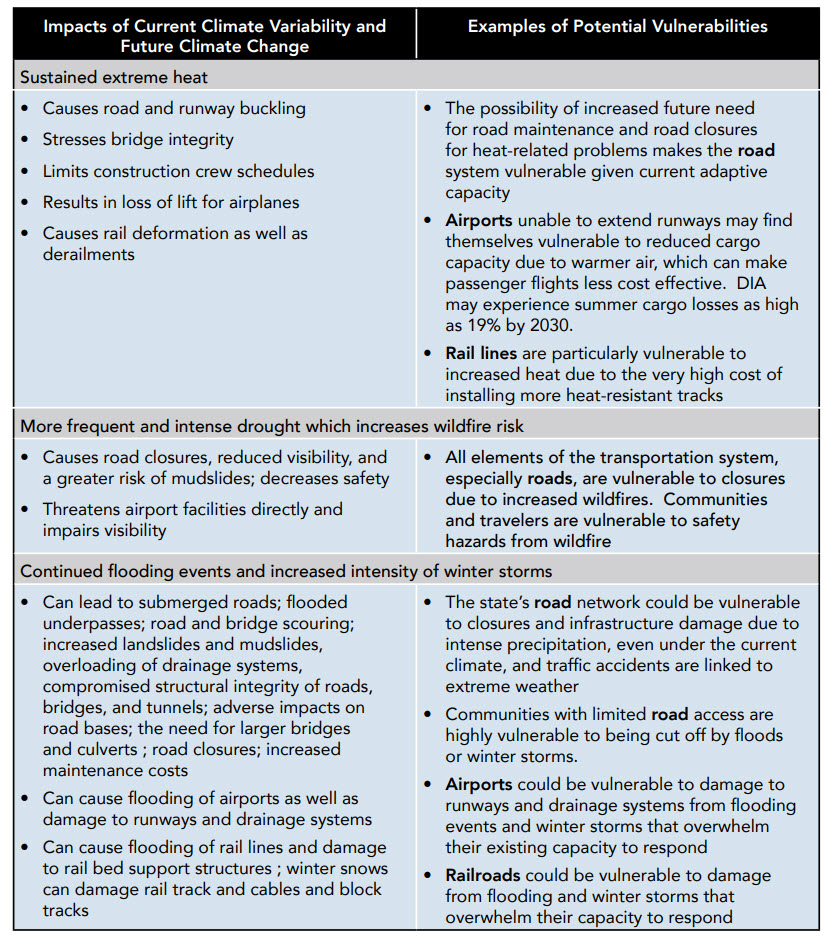
4 Impacts on Pavements
There is no national document or consensus on a full catalog of pavement impacts although several early efforts suggest what this might look like. Meyer et al. (2014) provide one of the more comprehensive lists of climate change impacts on pavements and potential adaptation strategies. In 2015, Muench and Van Dam (2015) modified and expanded that list, which describes the range of climate impacts relevant to pavements. There is a growing body of literature that addresses these climate change impacts on pavements, some of which also address associated adaptation strategies. Typically, these impacts and adaptations are addressed singularly rather than as a combined system. Table 2 is a summary of climate change impacts related to specific pavement impacts and potential adaptation strategies.
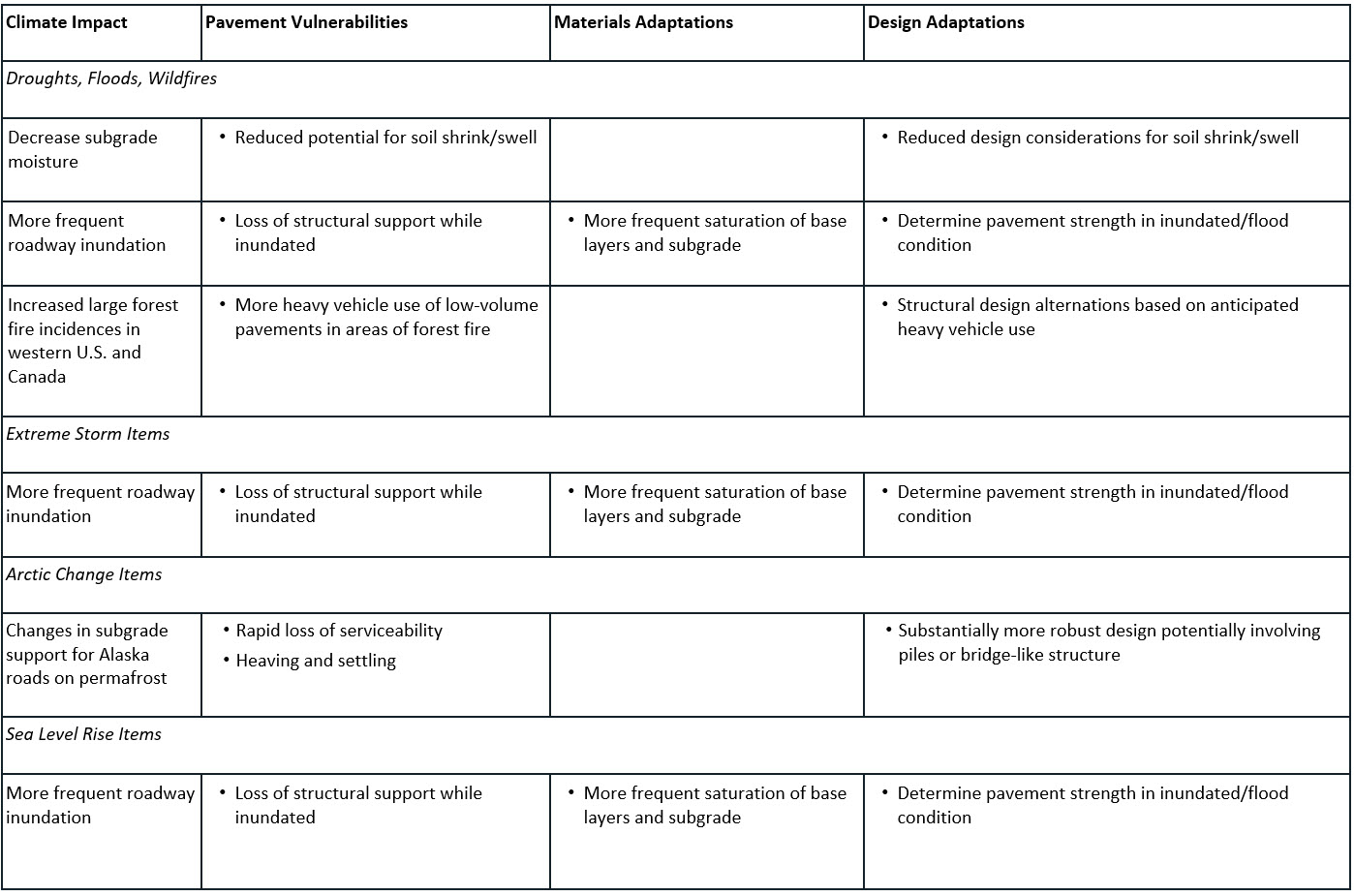
Willway et al. (2008a; 2008b) produced a similar list for climate change effects on pavements and mitigation efforts for the U.K. and concluded the three major impacts are temperature, precipitation, and soil moisture.
5 Resilience
A consensus resilience definition might best be represented by that proposed by the United Nations (UN 2016):
“The ability of a system, community or society exposed to hazards to resist, absorb, accommodate, adapt to, transform and recover from the effects of a hazard in a timely and efficient manner, including through the preservation and restoration of its essential basic structures and functions through risk management.”
This U.N. definition is appropriate at the system, community or society level. To focus more narrowly on the U.S. transportation system, FHWA Order 5520 defines resilience as:
“…the ability to anticipate, prepare for, and adapt to changing conditions and withstand, respond to, and recover rapidly from disruptions.”
This document uses the FHWA Order 5520 definition and focuses on how pavement systems contribute to transportation system resilience. Key characteristics of this resilience definition are:
- Resilience addresses both climate change adaptation and disaster risk reduction (Arup 2014). While longer-term climate change impacts are considered, current events often focus on extreme weather events (e.g., hurricanes, floods, droughts, heat waves), as well as other disaster events (e.g., earthquake, wild fire, tsunami).
- Resilience is a system characteristic. Resilience is an expression of how an entire system (e.g., road network) plans for, responds to, and recovers from changing conditions and disruptions. This implies it is a composite measure and is not specific to a single hazard or condition.
- Resilience must consider effects on interacting systems. Optimal resilience for one system may have detrimental effects on others including social and environmental systems. For instance, even though modern canals and levees in the Everglades may have contributed to improved flooding resilience, they also degraded the region’s hydrodynamic cycle, water quality, and ecology (Harvey et al. 2010).
- Resilience goes beyond traditional engineering qualities. While strength, durability, and function are important, resilience also considers finance, leadership, security, community support, public health and more (Arup 2014). For instance, it may be that materials and methods are available to improve a pavement’s resilience, but financing or the public will to do so is not.
- Resilience is not an absolute quality, but rather a quality spectrum. It is appropriate to discuss how resilient an infrastructure asset might be in relation to another or a set of metrics, but it is not possible to label something as “resilient” or “not resilient” as if it were an absolute measure.
- Resilience is a form of risk management. The actions associated with resilience align closely with traditional risk management actions: risk reduction, transfer/share risks, improving preparation, and responding and recovering effectively (Mitchell and Harris 2012).
5.1 Resilience Scope
Disruptions and change addressed by resilience in this document are limited to natural hazards (i.e., climate change) and their secondary stressors (e.g., emergency evacuation). Societal stressors such as conflict, human rights and welfare, and financial shock are not addressed. Defining resilience in this limiting way risks forgetting or de-emphasizing the “…role of agency, power, and politics” (Mitchell and Harris 2012) in system resilience. These societal attributes are often far more important in defining a community’s resilience to a disruption or change than technical abilities, materials characteristics, or design parameters.
5.2 Resilience in Relation to Design/Operating Parameters
Selecting appropriate design/operating parameters can address some resiliency issues. Traditionally, engineering design parameters are selected to ensure infrastructure function given a certain level of severity in the historic spectrum of environmental stressors; for instance, the water level associated with a 100-year recurrence interval for flooding or the peak ground acceleration associated with a 500-year recurrence interval earthquake. However, it may be that climate change has rendered historic climatic data obsolete as it can no longer be reliably used to predict future events, their severity, or their recurrence interval. Thus, data from historical records may have to give way to data from predictive models (in the case of climate) for use as design/operation input values.
Even with better design parameters, there is always a finite and accepted probability that infrastructure will be subject to stressors beyond those parameters and potentially fail (i.e., the disaster risk). In disaster situations, it is useful to quantify infrastructure response and plan for recovery since timely recovery may be required for society to respond to the disaster.
5.3 Quantifying Resilience
Resilience can be quantified but efforts to do so remain largely theoretical, discipline-specific, and fail to provide much in the way of meaningful solutions (Tamvakis and Xenidis 2013); still, work to develop a greater universal framework continues (Yodo and Wang 2016). Practical efforts (e.g., the Rockefeller Foundation’s City Resilience Index) tend to rely on indexes that use general or qualitative metrics, which seem to serve their purpose well.
5.4 Resilience Approach
5.4.1 General Approach
The climate change literature contains much direction and guidance in how to plan for, execute, and integrate resilience into organizations, functions, or topics. Among the most relevant for pavements include The U.S. Climate Change Resilience Toolkit (NOAA et al. 2015), the FHWA’s Climate Change Adaptation Guide for Transportation Systems Management, Operations, and Maintenance (Asam et al. 2015), the FHWA’s’ Synthesis of Approaches for Addressing Resilience in Project Development (Choate et al. 2017), and NCHRP Synthesis 527, Resilience in Transportation Planning Engineering, Management, Policy, and Administration (Flannery et al. 2018). Integrating these references, a general approach to resilience can be stated:
- Define scope.
- Define which assets are valued and how they are valued
- Identify key climate variables that may cause damage to them
- Assess vulnerability and risks
- Determine what assets are exposed to harm
- Gather appropriate climate data
- Assess vulnerability
- Estimate the risk to each asset
- Investigate options
- Consider possible solutions
- See what others have done
- Prioritize and plan
- Evaluate feasibility of actions
- Make a plan to implement the most feasible actions
- Take action
- Implement the plan
- Check to see if it improves resilience
5.4.2 Resilience Approach for Pavements
This section discusses the previous approach as it relates to pavements and what has been done so far.
- Define scope.
- The generic scope is defined as the pavement structure. This includes all supporting pavement layers down to but not including the untreated subgrade.
- Understand the context. This means the relative importance of the pavement: its critical function, amount of acceptable damage, or other characteristics. This context may be defined by high traffic volumes, higher-level prioritization of acceptable maintenance intervals, life-cycle costs, evacuation routes, or other criteria. There have been limited efforts to prioritize pavements specifically; usually efforts are at a city/region/DOT level for routes, routes, corridors and bridges only.
- Define future climate items that may impact the pavement; especially those that are different in type or magnitude from the present. This is a high-level investigation of which general climate change impacts may affect the pavement. Ideally, current climate conditions are already accounted for in pavement design, construction, operations and maintenance. Therefore, it is critical to highlight how conditions may change in the future to those that may not be accounted for in current design, construction, operations and maintenance. Broad transportation climate change and resilience assessments, such as by Muench and Van Dam (2015), have done reasonably well at identifying climate variables that may cause damage and assets that may be exposed to harm. Literature most commonly focuses on temperature and moisture changes as well as flooding effects.
- Assess vulnerability and risks.
- Gather appropriate climate data. Generally this means finding key climate data from predictive models that has been appropriately scaled down to the local level for use as inputs to models, design standards, or materials specifications. A number or research efforts have used downscaled climate data as inputs to performance models; usually AASHTOWare ME Pavement Design software. Determining appropriate climate data sets and how best to downscale them to a local level is still an active area of research.
- Assess vulnerability to climate-related changes or hazards. Determine which pavements are exposed (in areas where they are impacted), how sensitive they are (how are they affected), what their adaptive capacity is (ability to cope with impacts). Collectively, exposure, sensitivity, and adaptive capacity define vulnerability. Individual research efforts have done this for specific pavements, geographic areas, or hazards.
- Characterize the risk from climate impacts. This includes defining the probability and cost of predicted impacts. It may be that probability can best be characterized by a few generic statements such as those used by the USGCRP (2018): confidence levels of very high, high, moderate, and low; or likelihood from virtually certain (99-100%) down to exceptionally unlikely (0-1%). Most current research assumes a climate scenario (e.g., RCP8.5) and calculates, rather precisely, impacts based on modeling. There is little work to characterize compound risk, or the gradations of risk necessary to allow for decisions.
- Investigate options.
- Consider possible solutions. Investigate a wide range of actions. Be sure to include options that involve different budgets (high down to zero) and even partial solutions. Research to date generally assumes a few solutions (e.g., increase pavement thickness, change PG grade, reduce joint spacing) and then calculates the impact of those solutions. Current research is more apt to describe a few potential solutions and associated impacts rather than a full range of solutions or partial solutions. There is little research on options/actions to evaluate, prioritize, and restore pavements to service after damage.
- See what others have done. Learn from others. Some literature is emerging on climate change adaptation. For instance, there is a fair level of literature on the aftereffects of flooding on pavements from actual floods, and some communities have documented sea-level rise adaptation (e.g., South Beach, FL).
- Prioritize and plan.
- Evaluate feasibility of actions. Evaluate the efficacy of possible actions under future climate scenarios. Account for the context identified in Step 1. Determine whether actions can reduce risks and what trade-offs may be necessary in light of limited resources. Little work exists on how to systematically evaluate the feasibility of actions. Evaluating the efficacy of actions is generally limited to evaluating pavement design model outputs using predicted future climate inputs.
- Make a plan to implement the most feasible actions. A few communities have implemented initial plans for some climate change issues; most notably sea-level rise.
- Take action.
- Implement the plan. A few communities have implemented initial plans for some climate change issues; most notably sea-level rise.
- Check to see if it improves resilience. Measure the effectiveness of the plan and monitor results. Adjust accordingly. There is nearly no literature addressing the effectiveness of resilience measures.
5.5 Recent U.S. Directives on Transportation Infrastructure Resilience
Resilience, as defined in this document, has begun to appear in official documents and directives associated with U.S. transportation infrastructure. These directives are the clearest manifestation of U.S. society’s desire to address resilience. Here are a few of the more prominent mentions:
- U.S. DOT Strategic Plan for FY 2018-2022 (USDOT 2018). Strategic Objective 2 (Life Cycle and Preventive Maintenance) includes a statement that a State DOT “…will increase its effectiveness in ensuring that infrastructure is resilient enough to withstand extreme weather and security events (including cybersecurity events), which could otherwise disrupt the transportation network and require major reconstruction.”
- 23 CFR 667: Periodic Evaluation of Facilities Repeatedly Requiring Repair and Reconstruction Due to Emergency Events (FHWA 2016a). An FHWA mandate requiring state DOTs to periodically evaluate transportation facilities (roads, highways, bridges) that have required repair and reconstruction activities on two or more occasions due to emergency events and consider these evaluations when developing projects.
- FHWA Order 5520: Transportation System Preparedness and Resilience to Climate Change and Extreme Weather Events (FHWA 2014). This order establishes FHWA policy on preparedness and resilience to climate change and extreme weather events.
References
Arup International Development (ARUP). 2014. City Resilience Framework. Rockefeller Foundation, New York, NY. https://assets.rockefellerfoundation.org/app/uploads/20140410162455/City-Resilience-Framework-2015.pdf
Asam, S., C. Bhat, B. Dix, J. Bauer, and D. Gopalakrishna. 2015. Climate Change Adaptation Guide for Transportation Systems Management, Operations, and Maintenance. FHWA-HOP-15-026. Federal Highway Administration, Washington, D.C. https://ops.fhwa.dot.gov/publications/fhwahop15026/fhwahop15026.pdf
Childress, A., E. Gordon, T. Jedd, R. Klein, J. Lukas, and R. McKeown. 2015. Colorado Climate Change Vulnerability Study. University of Colorado and Colorado State University, Denver, CO. https://wwa.colorado.edu/climate/co2015vulnerability/co_vulnerability_report_2015_final.pdf
Federal Highway Administration (FHWA). 2014. Transportation System Preparedness and Resilience to Climate Change and Extreme Weather Events. Order 5520. Federal Highway Administration, Washington, DC. https://www.fhwa.dot.gov/legsregs/directives/orders/5520.cfm
Federal Highway Administration (FHWA). 2016a. Periodic Evaluation of Facilities Repeatedly Requiring Repair and Reconstruction Due to Emergency Events. 23 CFR, Part 667. Federal Register, Volume 81, No. 205. U.S. Department of Transportation, Washington, DC.
Federal Highway Administration (FHWA). 2017. Synthesis of Approaches for Addressing Resilience in Project Development. FHWA-HEP-17-082. Federal Highway Administration. Washington, DC. https://www.fhwa.dot.gov/environment/sustainability/resilience/ongoing_and_current_research/teacr/synthesis/fhwahep17082.pdf
Flannery, A. M. A. Pena, and J. Manns. 2018. Resilience in Transportation Planning, Engineering, Management, Policy, and Administration. NCHRP Synthesis 527. Transportation Research Board, Washington, DC.
Harvey, R. G., W. F. Loftus, J. S. Rehage, and F. J. Mazzotti. 2010. Effects of Canals and Levees on Everglades Ecosystems: Circular. WEC304. University of Florida Institute of Food and Agricultural Sciences (IFAS) Extension, Gainesville, FL.
Intergovernmental Panel on Climate Change (IPCC). 2014. Climate Change 2014: Synthesis Report. Contribution of Working Groups I, II and III to the Fifth Assessment Report of the Intergovernmental Panel on Climate Change [Core Writing Team, R.K. Pachauri and L.A. Meyer (eds.)]. IPCC, Geneva, Switzerland.
https://ar5-syr.ipcc.ch/ipcc/ipcc/resources/pdf/IPCC_SynthesisReport.pdf
Meyer, M., M. Flood, J. Keller, J. Lennon, G. McVoy, C. Dorney, K. Leonard, R. Hyman, and J. Smith. 2014. Strategic Issues Facing Transportation, Volume 2: Climate Change, Extreme Weather Events, and the Highway System. NCHRP Report 750. Transportation Research Board, Washington, D.C.
Mitchell, T. and K. Harris. 2012. Resilience: A Risk Management Approach. Overseas Development Institute, London, U.K.
Muench, S. and T. Van Dam. 2015. Climate Change Adaptation for Pavements. FHWA-HIF-15-015. Federal Highway Administration, Washington, DC. https://www.fhwa.dot.gov/pavement/sustainability/hif15015.pdf
Tamvakis, P. and Y. Xenidis. 2013. “Comparative Evaluation of Resilience Quantification Methods for Infrastructure Systems.” Procedia – Social and Behavioral Sciences, Volume 74. Elsevier BV.
United Nations (UN). 2016. Report of the Open-Ended Intergovernmental Expert Working Group on Indicators and Terminology Relating to Disaster Risk Reduction. United Nations Office for Disaster Risk Reduction, United Nations General Assembly, New York, NY. https://www.preventionweb.net/files/50683_oiewgreportenglish.pdf
U.S. Department of Transportation (USDOT). 2018. Strategic Plan for FY 2018-2022. U.S. Department of Transportation, Washington, DC. https://www.transportation.gov/sites/dot.gov/files/docs/mission/administrations/office-policy/304866/dot-strategic-planfy2018-2022508.pdf
USGCRP. 2017. Climate Science Special Report: Fourth National Climate Assessment, Volume I [Wuebbles, D.J., D.W. Fahey, K.A. Hibbard, D.J. Dokken, B.C. Stewart, and T.K. Maycock (eds.)]. U.S. Global Change Research Program, Washington, DC. https://science2017.globalchange.gov/
USGCRP. 2018. Impacts, Risks, and Adaptation in the United States: Fourth National Climate Assessment, Volume II: Report-in-Brief [Reidmiller, D.R., C.W. Avery, D.R. Easterling, K.E. Kunkel, K.L.M. Lewis, T.K. Maycock, and B.C. Stewart (eds.)]. U.S. Global Change Research Program, Washington, DC. https://nca2018.globalchange.gov/downloads/NCA4_Report-in-Brief.pdf
Willway, T., L. Baldachin, S. Reeves, M. Harding, M. McHale, and M. Nunn. 2008a. The Effects of Climate Change on Highway Pavements and How to Minimise Them: Technical Report. PPR184. Transport Research Laboratory, United Kingdom.
Willway, T.; Reeves, S. and Baldachin, L. 2008b. Maintaining Pavements in a Changing Climate. Transport Research Laboratory, United Kingdom.
Yodo, N. and P. Wang. 2016. “Engineering Resilience Quantification and Design Implications: A Literature Survey.” Journal of Mechanical Design, Volume 138, No. 11. American Society of Mechanical Engineers, New York, NY.